Research Motivation
The self-organization of many simple and deformable elements creates a rich variety of physical adaptation in nature in a bottom-up manner. These examples exist on multiple scale lengths; from embryonic cells to fish schools. Conversely, we follow a top-down approach to build robotic mechanisms to realize predefined designs that are optimized for certain tasks and environments.
I believe that the novel physically adaptive robots exist in the space that combines these two complementary design approaches. While soft deformable materials and self-assembling systems allow us to explore unprecedented robotic forms and functions in a bottom-up manner, top-down control, automation and machine learning methods enable repeatability and programmability of these novel systems. My research focuses on developing the tools and platforms to investigate this search space.
I am a “hands-on” robotics researcher and engineer; meaning that I design, build, program, and analyze robotic systems, commanding over both hardware and software components.
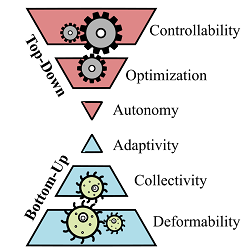
My research areas
1 – Soft Robotics
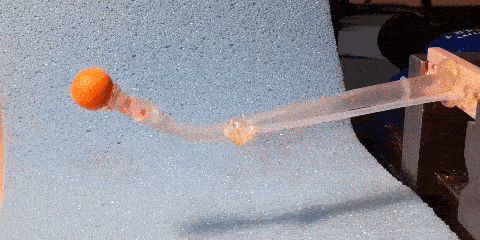
The depiction of a robot used to be not much different than an aluminum toaster for a long time. Inspirations from biology changed this outlook and introduced soft and deformable materials to robot designs. With the advances in materials research, now we can easily fabricate and functionalize such materials and build robots that perform tasks, which are not possible for conventional robots. I benefit from the viscoelastic deformation of soft materials to build robots that have application potential in manipulation, sensing, and locomotion.
Adaptive Manipulation
Manipulation (not to be confused with the synonym term in psychology) is one of the fundamental functions for survival and adaptation in nature. From the most simple to the most complex, organisms have evolved certain body appendages to perform manipulation tasks that define their physical adaptation capabilities. It is no surprise that the understanding and analysis of this function has become one of the basic elements of robotics science, and my research. Taking the human hand as the template, I am specifically interested in the design and development of robotic manipulators that are both dexterous and physically compliant. I achieve this goal by combining rigid and soft materials, and applying machine learning methods to find optimum design and control parameters.
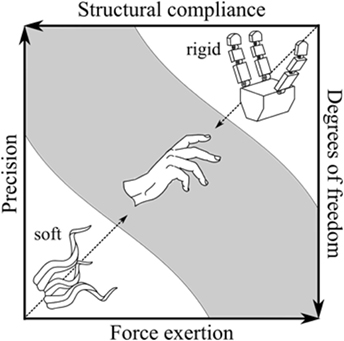
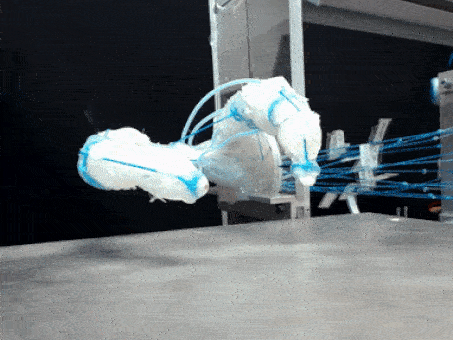
The common principles of physically adaptive manipulators establish a balance between damping, forces, precision, and compliance.
Publications:
The hybrid use of hard and soft materials allows the robotic manipulators to perform anthropomorphic tasks.
Publications:
Soft Sensing
Just like manipulation, sensing is crucial for physical adaptation as it provides vital information about the changes that are constantly happening outside and inside an organism, and naturally a robot. My research focuses on the soft robotics sensing perspective, where I address the challenges in gathering tactile information from continuous and deformable robot bodies, and finding clever solutions to correlate visual information to soft robot kinematics.
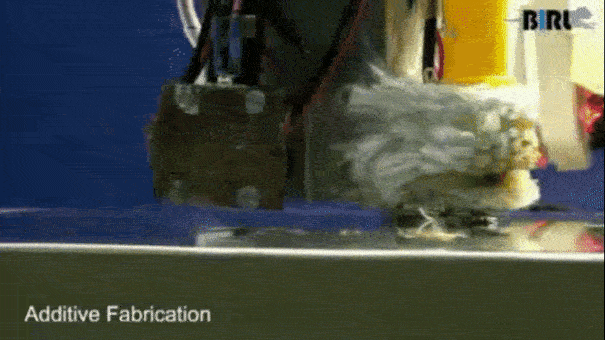
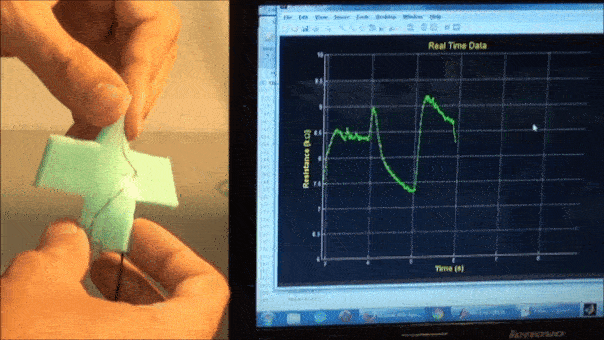
The in situ fabrication of soft sensors makes it possible for the robots to actively sense the stimuli in their environment.
Publications:
A functional conductive thermoplastic material allows the tactile sensing of dynamic deformations on a soft continuum surface.
Publications:
Multi-terrain Locomotion
Organisms and robots need to move from one place to another for different reasons, but the role of locomotion in physical adaptation is the same for both kingdoms. From the soft robotics perspective, the soft body elements used for locomotion may enable creative means of motion, but the control of the whole process becomes challenging compared to conventional rigid body dynamics systems. My research focuses on designing the compliant robotic locomotion appendages and exploring their efficient and adaptive control architectures.
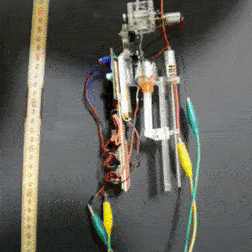
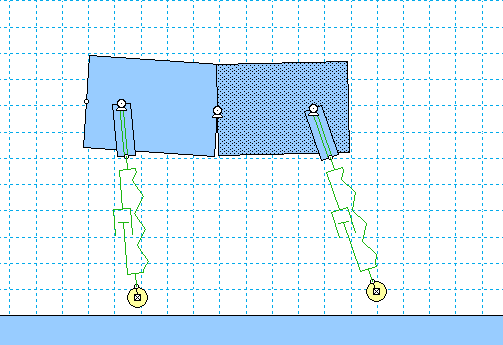
Inspired from spiders, the in situ fabrication of a dragline with soft materials allows free space locomotion.
Publications:
The compliant musculoskeletal system enables efficient and adaptive terrestrial locomotion both for animals and robots.
Publications:
- Myorobotics project in the International Symposium on Adaptive Motion of Animals and Machines (2013),
- Int. Conf. on Robotics and Automation – ICRA (2011).
2 – Machine Learning
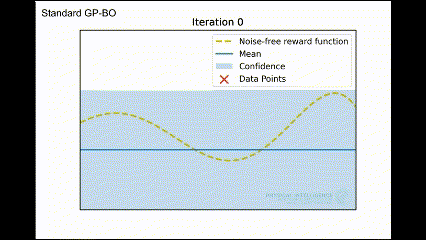
Soft robots hold promise for physical adaptation through viscoelastic deformations, but their flexibility and material-driven behavior make it difficult to model, predict, and control their movements using conventional methods. Common approximation techniques, like body discretization and constant curvature assumptions, often fall short.
I believe that advanced machine learning can help us better explore and understand the design and functionality of soft robots. My work focuses on applying machine learning to learn these complex models and developing platforms for reliable experiments on physical systems. I extend this application to other complex processes without models as well.
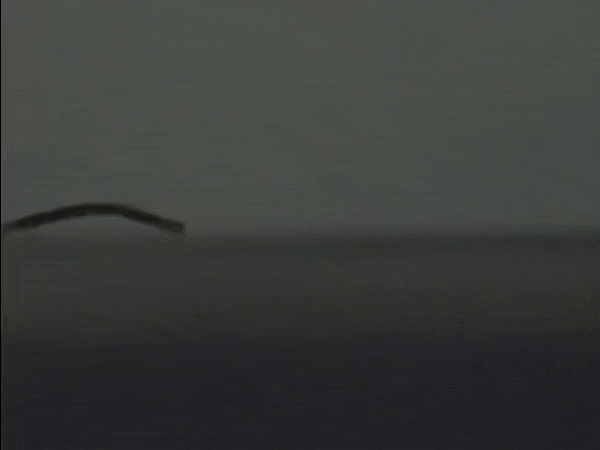
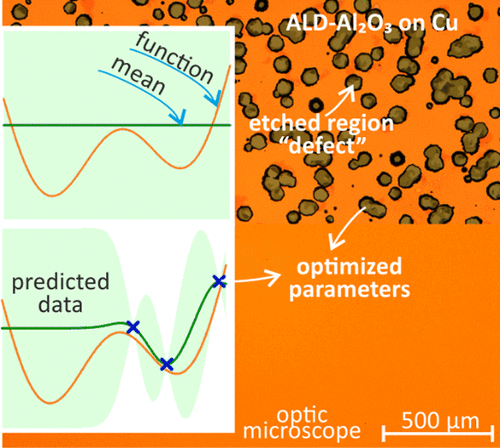
Learning the controller parameters of a miniature soft robot using Bayesian Optimization and Gaussian Processes.
Publications:
Optimizing the fabrication parameters of the Atomic Layer Deposition of thin films for chip manufacturing.
Publications:
3 – Self-Assembly
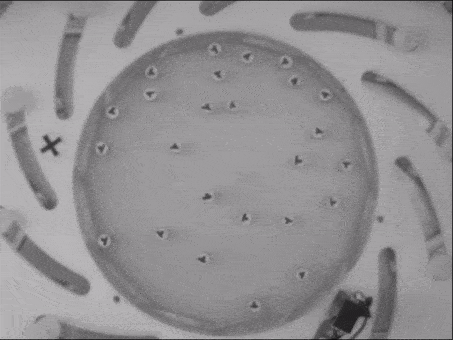
Self-assembly is a ubiquitous process in both the living and non-living systems that generate complex and functional structures from the local interactions between a large set of simpler components. Especially in biology, physical adaptation is explained by linking the emergence of functions to the formation of such structures as a result of the collective behavior of neighboring cells.
The ability to control the self-assembly process is very exciting for the robotics field as it may enable alternative design methods to build novel robotic systems. This is also an interdisciplinary line of research that will additionally elucidate fundamental physics and enable the fabrication of novel functional materials. My research focuses on the design of physical mechanisms that allow the programming of the self-assembly pathway of robotic systems.
Publications: